Being small is good. Being very small is better. That is the philosophy of Mark Kester, University of Virginia professor of biomedical engineering, pharmacology and molecular physiology and biophysics.
Kester’s research focuses on particles that are smaller than 100 nanometers, known as nanoparticles, which can be engineered to hold new properties that cannot be explained by conventional physics. By exploiting these properties – which is the mission of UVA’s nanoSTAR Institute – nanotechnology can produce more effective drugs and vaccines, faster computers, better batteries for the next generation of electric cars, lighter airplane wings and countless other possibilities.
Kester directs the institute, which falls under UVA’s School of Engineering but is a collaborative research effort that incorporates faculty from across Grounds.
This summer, the institute added another layer to its Summer Undergraduate Research Program, which historically has placed rising second-year students in nanotechnology labs that match their academic interests. After a year’s delay due to the pandemic, Kester and the institute curated the nanoSTAR Summer Program for Entrepreneurial Nanoscale Engineering. With the help of Dr. Bernie Carlson, director of UVA’s engineering business programs, and backed by a $100,000 grant from the Jefferson Trust, nine students received the opportunity to develop their own intellectual property while learning the entrepreneurial skills to eventually bring it to the market.
Third-year physics and psychology major Ziyuan Wang was one of those students. Through her nanoscale research, under the supervison of Dr. Bellave Shivaram , Associate Professor of Physics, Wang plans to explore the special behaviors of superfluidity – a state of matter that is neither gas, liquid nor solid – using superfluid helium droplets. With zero viscosity and the ability to flow infinitely, Wang describes the matter as magical, as it can creep up walls and flow through containers undisturbed.
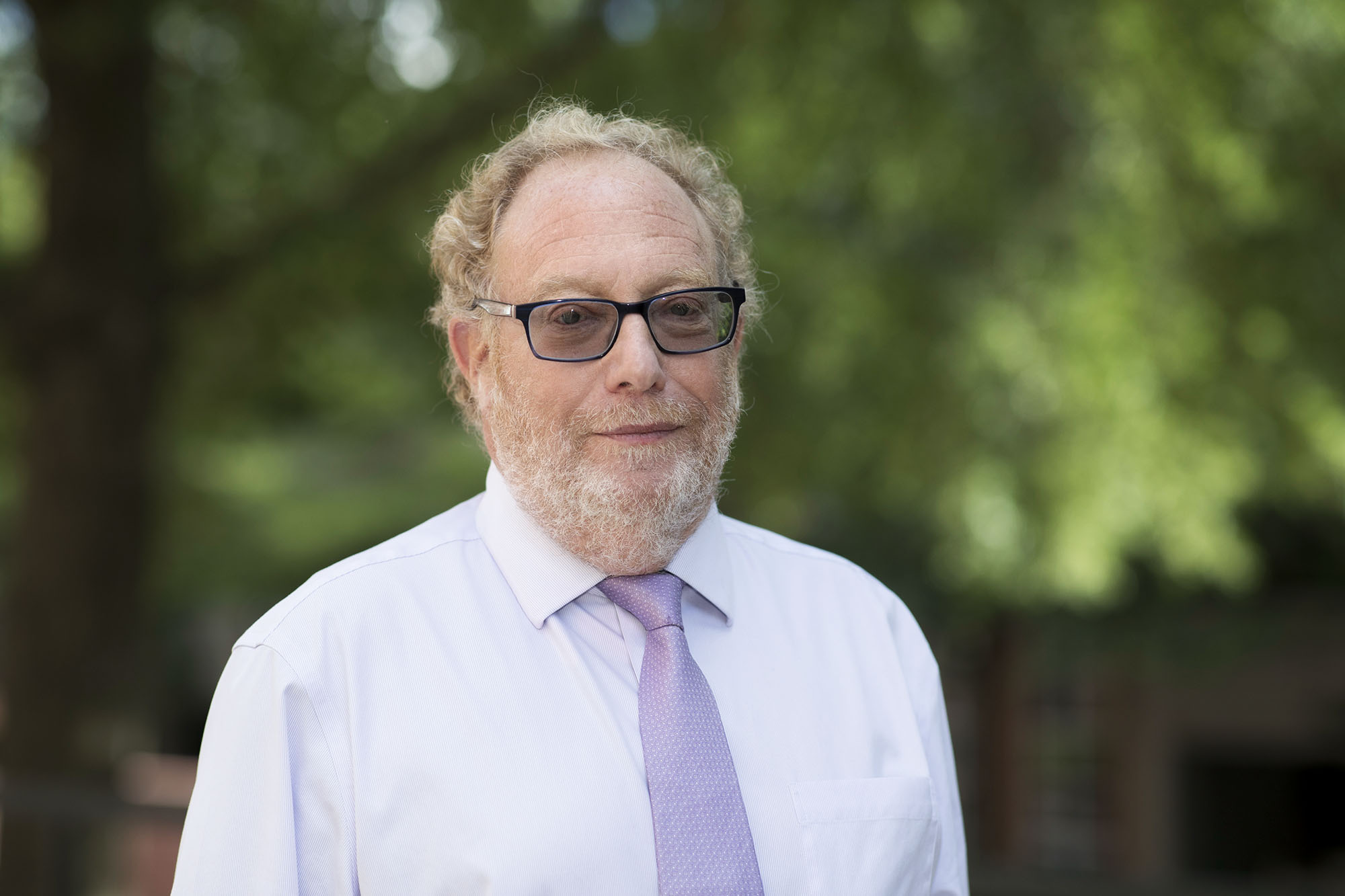
UVA professor of biomedical engineering, pharmacology and molecular physiology and biophysics, Mark Kester, is also the director of the nanoSTAR Institute. (Photo by Dan Addison, University Communications)
“We are studying superfluid, like a lot of labs around the world, because it is a really good model of the quantum world,” Wang said. “This project can someday get us more insights on properties of the quantum world that we can observe in a macroscopic way.”
(Superfluid may also help explain the origins of the universe, but more on that later.)
Before Wang, alongside her two undergraduate colleagues Maxwell Wisnieski and Rose Knight, can explore the special properties of superfluid helium, they first had to engineer an apparatus by which these nanoscale droplets could be observed. This came in the form of a metal vacuum chamber containing a window coated in a thin cesium layer only a few atoms in width. At the start of the summer program, Wang began assembling the chamber while her teammates began developing a laser beam controller system and power supply to run the apparatus. By early September, the group had completed their separate tasks, and will soon begin using the laser system to draw patterns on the cesium surface.
“Coming in, I had no idea what a vacuum chamber even looked like,” Wang said. “You have to check a lot of data sheets and manuals to see how everything works, since nothing we got came with very specific instructions. … But we figured it out along the way.”
Since cesium reacts violently with both oxygen and water, the group’s biggest challenge was dropping the vacuum to a low enough pressure, to the point where neither oxygen nor water was left inside. Many screws and five vacuum pumps later, Wang was able to hit a low enough pressure by wrapping the vacuum in heating strips for a slow, eight-hour bake up to 150 degrees Celsius.
“In our next step, we will be placing cesium dispensers into the vacuum and forming a thin layer of cesium onto this mirror,” Wang said. “We will then direct lasers on it to draw images, to make sure we can later manipulate helium droplets on the surface.”
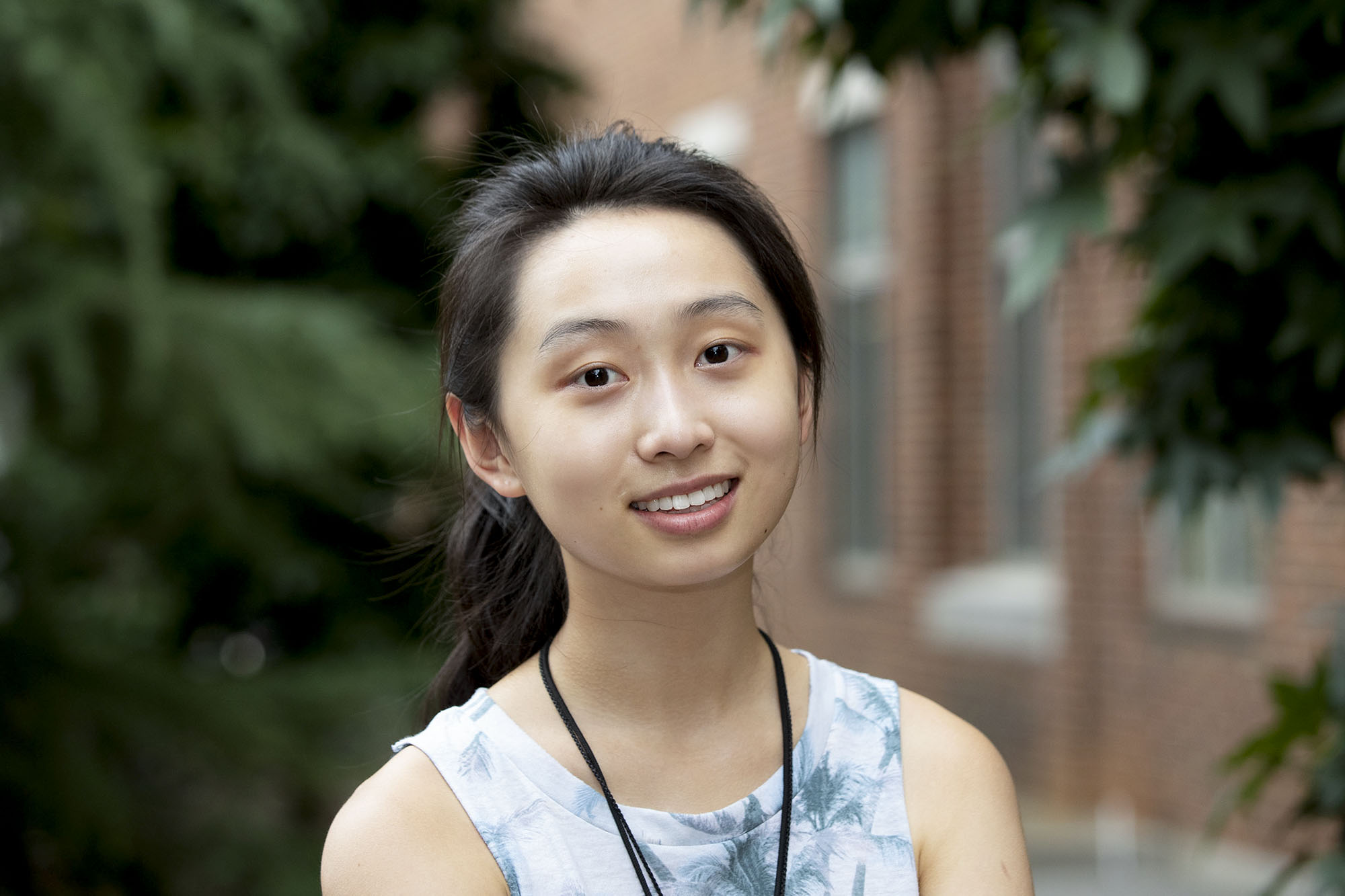
Wang was one of nine students participating in the nanoSTAR Summer Program for Entrepreneurial Nanoscale Engineering, working to commercialize nanotechnology innovations. (Photo by Dan Addison, University Communications)
Rather than having an end product that can be directly commercialized, Wang explained that the cesium coating they are working on has applications in many fields, from coatings on eyeglass lenses and furniture surfaces to military machinery and energy-efficient solar panels. Additionally, the knowledge that they hope to gain may create insights to advance the prospect of quantum computing — a computational method that surpasses supercomputers, with the ability to analyze mass amounts of data to solve complex problems and find optimal combinations.
When it comes to explaining the origins of the universe, two findings have led to theories that place superfluid helium at the beginning. The first is that unlike normal fluids that have one, tornado-like vortex when spun, superfluid helium creates multiple vortices. The second is that the universe had a uniform density at its beginning, just like superfluid.
“Scientists have theorized that these vortices that are present when you spin a superfluid droplet may be how the first galaxies were formed in a universe,” Wang said. “That is something we might be able to observe in the future.”
While Wang was working on nanomaterials, third-year biochemistry major and Army ROTC cadet Christopher Nguyen used the eight-week summer program to advance a nanomedicine project called Manifold. As a member of the Virginia iGEM Team, directed by UVA Biology Professor, Dr. Keith Kozminski,, the group is developing a biosynthetic device they hope will help alleviate drug shortages, specifically for life-saving, complex drugs.
For their proof of concept, the group is using the over-the-counter heart medication, resveratrol. Since drugs of similar complexity cannot be synthesized as efficiently as simpler ones, such as aspirin, many pharmacies do not store them, often resulting in restricted access for patients.
The shortage issue stems from the lengthy and inefficient processes of plant-then-chemical synthesis, by which complex drugs are primarily produced. Plant synthesis requires lots of space and resources, while chemical synthesis consists of extensive reactions and purification steps, producing low yields and high impurities.
To counter these inefficiencies, Manifold is introducing a more sustainable approach to prokaryotic biosynthesis – the process by which bacterial cells “grow” a desired drug – to the pharmaceutical industry. Unlike plant and chemical synthesis, biosynthesis places drug-producing bacteria in bioreactors in which temperature, pH and growth medium can be controlled for large volumes of cells. With higher temperatures and ideal growth conditions, the rate of biosynthesis increases, allowing these bacteria to mass produce the desired drug.
“Cells are very special in that they’ve undergone billions of years of evolution, which allows them to make complicated structures very efficiently,” Nguyen said. “Many of these complicated drugs are already encoded in their genome. All you have to do is figure out some way to mass-produce those drugs moreso than what [bacterial cells] normally do.”
To make an even more efficient biosynthetic process, Nguyen’s group is tackling four issues, all of which arise from the fact that biosynthesis occurs in the cytosol, or liquid interior, of bacterial cells. With such a large reaction space, biosynthesis encounters loss of intermediates and flux imbalance, where intermediates diffuse anywhere in the cell and prevent reactions; as well as pathway competition, in which intermediates are used in the wrong reactions to produce undesired products. Additionally, generating toxic products is quite common, and can harm or even kill the bacterial cell due to the lack of natural compartmentalization. All of these problems inhibit industrial biosynthesis.
Utilizing special organelles called bacterial microcompartments, found only in specific types of bacteria, Nguyen’s group is engineering artificial DNA scaffolds that localize certain enzymes inside these organelles to solve the four problems of biosynthesis. By using bacterial microcompartments, which are 40 to 200 nanometers in diameter, biosynthesis reactions occur in a smaller, more organized space; intermediates are sequestered from the cytosol, toxic substances are encapsulated inside the organelle and the inability for intermediates to diffuse outside the microcompartments prevents pathway competition.
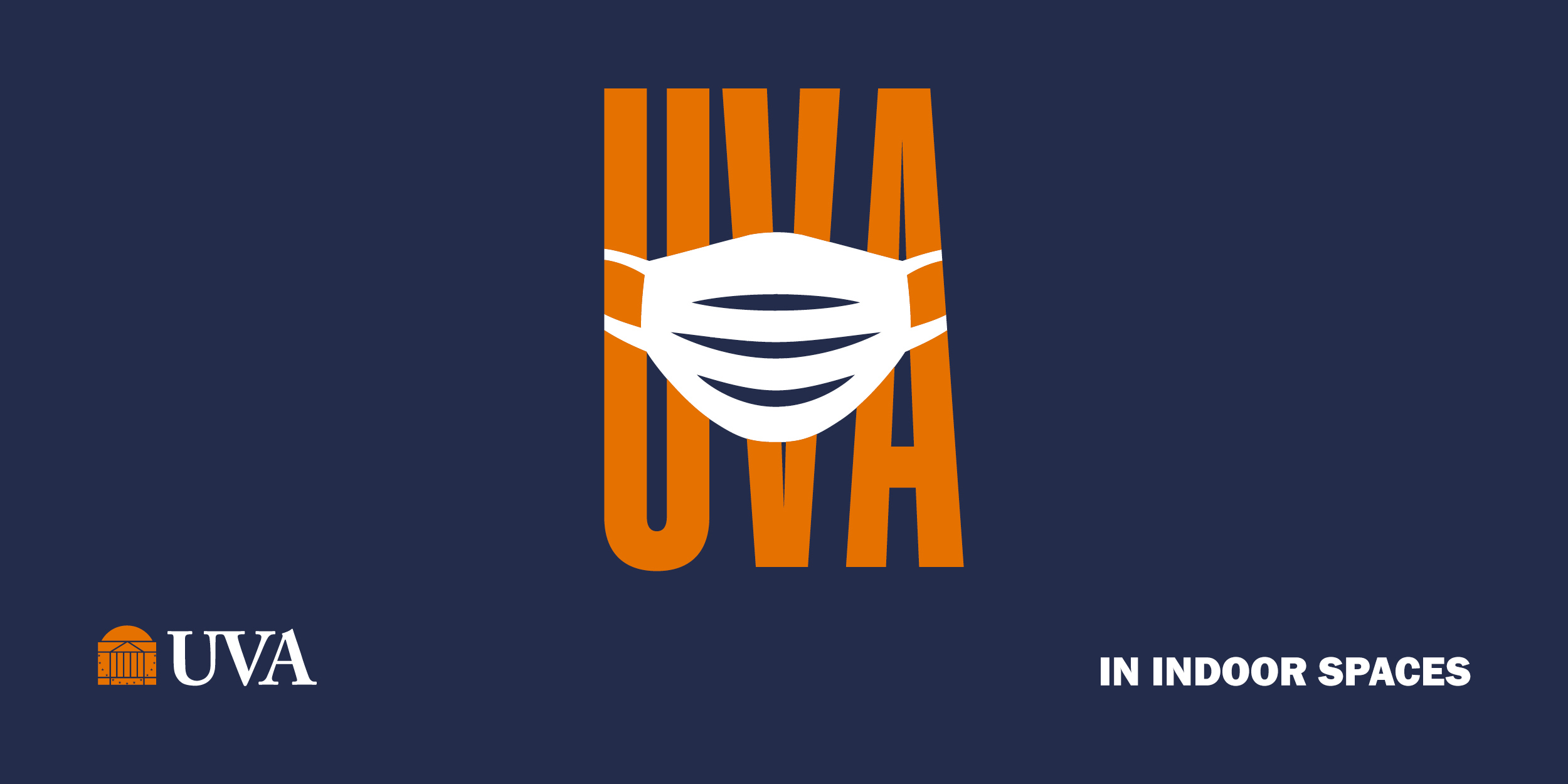
“Essentially, we are trying to create a bacterial cell that can sustainably produce all the pharmaceuticals needed in modern medicine without being limited by the four existing problems to biosynthesis,” Nguyen said.
After creating their proof of concept by synthesizing resveratrol in E. coli, Manifold has received a provisional patent, and is now filing for a full patent. In November, the Virginia iGEM Team will present their research and represent the University of Virginia at the international synthetic biology competition called the Giant Jamboree.
“This is a new synthetic biology platform,” Nguyen said. “No company has really done this, and it solves so many problems in pharmaceutical manufacturing and medicine, while introducing future applications in agriculture biotechnology, chemical manufacturing, biofuels and nanotechnology. I’m excited to see where this technology goes.”
In the next iteration of the nanoSTAR entrepreneurial program, the institute hopes to fund 30 to 40 students, rather than nine, and expand to invite historically black colleges and universities in the mid-Atlantic region to participate.
“The project spans the breadth and depth of nanotechnology here at the University,” Kester said. “Bottom line, that is what we want to do … get cool ideas, work with faculty, and turn them into business plans that could be exploited into companies.”
Media Contact
Article Information
September 20, 2021
/content/magical-superfluid-synthetic-biology-students-excel-nanotechnology