We are all pretty familiar with how our bodies sense what is going on in the outside world – what we see, hear, touch, taste or smell.
But exactly how do our brains sense and react to what is going on internally – pain, or hunger, or the simple need to breathe?
This internal sense is called interoception, and it’s often referred to as the body’s “sixth sense.” The concept has been around for a while, but neuroscientists have grown even more interested as technological and scientific breakthroughs allow us to more precisely understand how our bodies relay signals from an organ to the brain and back again.
“We have more tools to bring to bear, and more recognition that these internal sensor systems have not been as well-studied as the five senses we all know,” said neuroscientist Doug Bayliss, professor and chair of the University of Virginia Pharmacology Department. “Interoceptive systems should get equal attention, because they have important consequences for everything from how we breathe to how we control eating, sleep, blood pressure or pain.”

Bayliss is a member of UVA’s Brain Institute, which is building a network of scientists across Grounds focused on some of the most important problems in neuroscience today. We asked him to tell us more about interoception.
Q. How do you define interoception?
A. Typically, when we think of our sensory systems, we think of sensing things outside the body. Interoception is sensing things inside the body. It encompasses a wide range of sensory systems. Some are conscious, sending signals that we act on volitionally – you feel hungry and you eat; you feel tired and you sleep. Others are unconscious, such as the homeostatic systems that sense a spike in blood pressure and instruct your body to lower your heart rate, helping your blood pressure return to normal. One example that has been of interest during COVID-19 relates to low blood oxygen levels. We don’t really understand how respiratory insufficiency leads to the unpleasant internal sensation of “air hunger” (so-called dyspnea) and why COVID patients often do not present with this symptom despite sometimes dangerously low oxygen levels.
We have known about some of these systems for a while, but there are also some that we are just now discovering or understanding.
Q. How is new technology increasing interest in this area of study?
A. In some ways, the word “interoception” is a rebranding of something that scientists have studied for a long time. However, there is increasing interest because there are a lot of new tools available to help researchers explore our internal sensory systems.
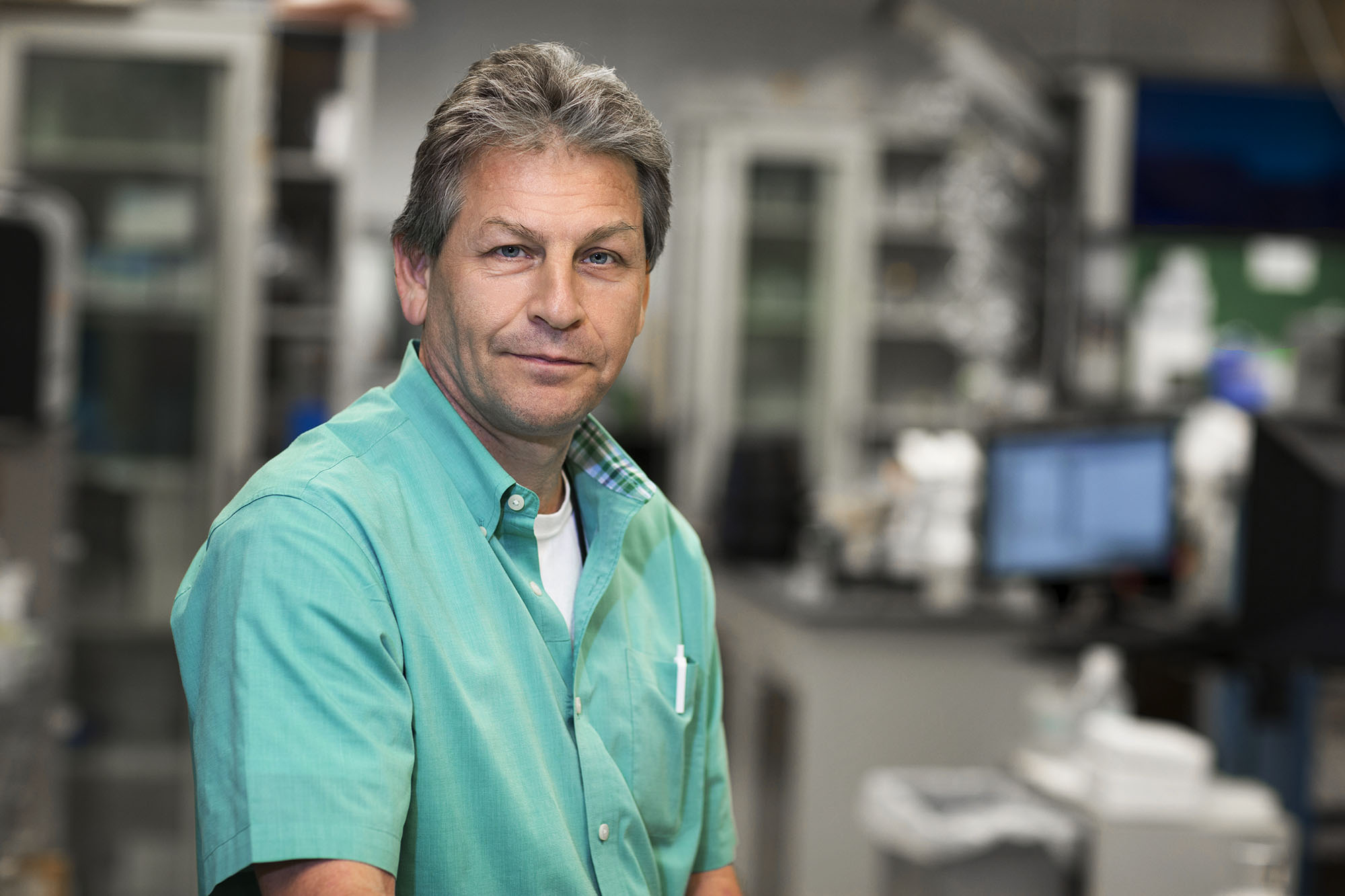
Doug Bayliss is a professor and chair of UVA’s Pharmacology Department. (Photo by Dan Addison, University Communications)
New molecular and genetic sequencing tools can help us identify exactly which classes of cells are driving a particular sensation. For example, we can identify the neurons with stretch-activated sensors in the lungs that convey to the brain when the lungs are expanding. Those cells can be identified by specific genetic markers, and we can record and follow their activity and behavior.
The next question is, are there ways we can control the activity of those cells? Can we make the brain think the lung is expanding even when it is not? Could that help us understand internal sensations associated with breathing (i.e., air hunger) and treat different disorders or illnesses? Similar approaches can be applied to many systems.
Q. Your research particularly focuses on how the body controls breathing. What have you learned?
A. One of the major control points for breathing is the level of carbon dioxide in your body. If you produce more CO2 because of a metabolic increase, then you need to breathe it out. There are sensors in the brain that detect elevated levels of CO2, which are connected to the motor systems that make your lungs breathe in and out. It is a homeostatic system that adjusts to keep CO2 levels at a stable point – similar to how you set the temperature in your house and then your air conditioner or furnace adjusts accordingly.
My lab, in collaboration with [recently retired UVA pharmacology colleague] Patrice Guyenet, is interested in identifying and tracking the brain cells that sense CO2 and the actual molecules in those cells that serve as detectors.
These cells are required for normal breathing, and studying them has clinical implications. For example, abnormalities in these cells likely contribute to a condition called congenital hypoventilation syndrome, a thankfully rare disorder that causes patients to stop breathing and fail to arouse during sleep. Obviously, this is an extremely dangerous condition; many patients have to be on a ventilator when they sleep.
Animal models of this disease show that the particular neurons we have been studying fail to develop. Understanding how these neurons affect sensory systems – interoceptive processes – can help us shed light on what these patients are experiencing and how we can help them.
Q. Where do you see the field going next?
A. There is a ton of interesting work going on at UVA in these areas. Ali Güler and John Campbell in the biology department are studying hunger. Researchers in the Center for Brain Immunology and Glia are understanding how our immune system senses pathogens and affects brain function.
Signaling also goes in the opposite direction; for example Patrice Guyenet and Dr. Mark Okusa are looking at how the brain controls immune system function. Many people are also doing fascinating research on the microbiome – the bacteria in our gut and how those bacteria affect our health. [UVA researchers have connected the microbiome to numerous health concerns.]
Interoception is a broad field that covers so many things, but it also provides a framework for developing collaboration between different research groups, building connections and studying interactions. There is broad national interest in the topic right now, and new technologies and discoveries are attracting a broader swath of neuroscientists. It’s exciting.
Media Contact
Article Information
April 9, 2021
/content/qa-what-interoception-and-why-are-neuroscientists-so-fascinated-it