If the amount of energy needed for computing keeps climbing, in just eight years up to 20% of the world’s power could be consumed by data centers, wireless networks, consumer electronics and a growing number of other devices.
That prediction from the company Enerdata – which pegs today’s computing energy consumption at up to 9% of the world’s power – is one reason researchers at the University of Virginia’s School of Engineering and Applied Science are working to make computing more energy efficient. That projected growth, coupled with power grids already under strain from weather-related events and the economy transitioning from fossil fuel to renewables, has engineers desperately trying to flatten computing’s energy-demand curve.
Members of Jon Ihlefeld’s multifunctional thin film group are doing their part. They are investigating a material system that will allow the semiconductor industry to put both memory and computation on a single chip.
“Right now we have a computer chip that does its computing activities with a little bit of memory on it,” said Ihlefeld, associate professor of materials science and engineering and electrical and computer engineering.
Every time the computer chip wants to talk to the larger memory bank, it sends a signal down the line, and that requires energy. The longer the distance, the more energy it takes. Today the distance can be up to several centimeters which, in computing distances, is really far.
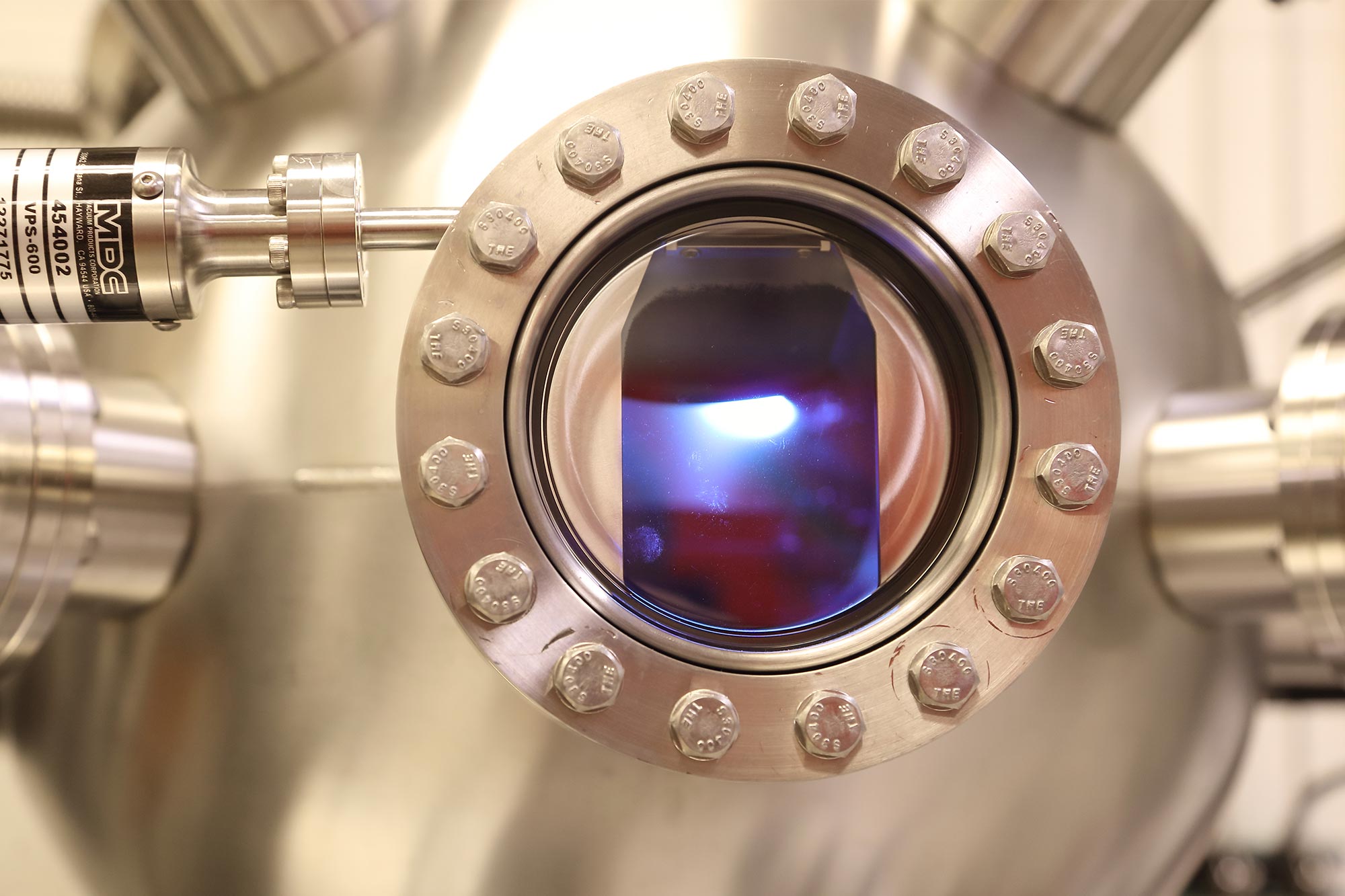
“In a perfect world, we would get them in direct contact with each other,” Ihlefeld said.
That requires memory materials that are compatible with the rest of the integrated circuit. One class of materials suitable for memory devices are ferroelectrics, meaning they can hold and release a charge on demand. However, most ferroelectrics are incompatible with silicon and do not perform well when made very small, a necessity for modern-day and future miniaturized devices.
Researchers in Ihlefeld’s lab are playing matchmaker. Their research advances materials with electrical and optical properties that make modern computation and communication possible. They also specialize in fabrication and characterization of a range of materials.
Their material of interest is hafnium oxide, which is used in the manufacture of cell phones and computers today. The downside is that in its natural state, hafnium oxide is not ferroelectric.
Over the last 11 years, it has become known that hafnium oxide’s atoms can be manipulated to produce and hold a ferroelectric phase, or structure. When a hafnium oxide thin film is heated, a process called annealing, its atoms can move into the crystallographic pattern of a ferroelectric material; when the thin film is cooled, its crystalline structure sets in place.
The process is complicated, but the result is a sandwich of sorts – the substrate, thin film and electrode – that becomes a capacitor.
Why formation of the ferroelectric phase happens has been the subject of much speculation. Shelby Fields, who earned a doctorate in materials science engineering from UVA this year, published a landmark study to explain how and why hafnium oxide forms into its useful, ferroelectric phase.
Fields’ work illustrates how to stabilize a hafnium oxide-based thin film when it is sandwiched between a metal substrate and an electrode.
“Now we understand why the top layer is such an important consideration. Down the line, people who want to integrate computing and memory on a single chip will have to think about all the processing steps more carefully,” Fields said.
Samantha Jaszewski is already thinking about that.
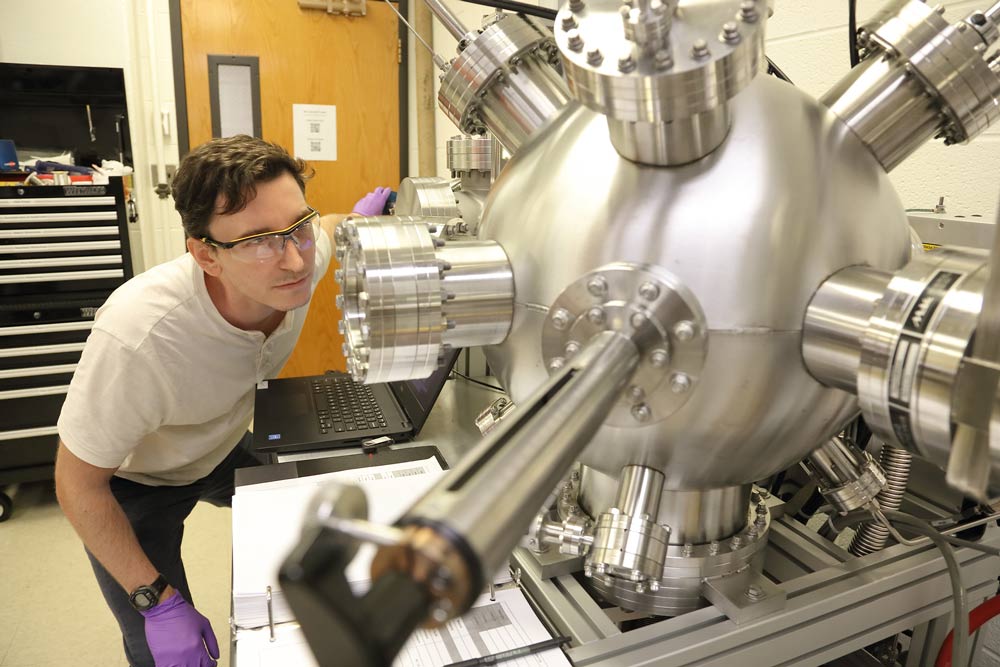
Jaszewski is a doctoral student of materials science and engineering and a member of Ihlefeld’s multifunctional thin film research group. She also wants to understand what contributes to the stability of hafnium oxide’s ferroelectric phase and how chip designers can control the material’s behavior.
The promise of this research is to create a chip that does double duty, which will slash power demands. The studies could help transform the semiconductor industry.
“These landmark studies explain why ferroelectric hafnium oxide exists and how it stabilizes,” Ihlefeld said. “Based on these new findings, we can engineer hafnium oxide thin films to be even more stable and perform even better in an actual application. By doing this fundamental research we can help semiconductor firms understand the origin of problems and how to prevent them in future production lines.”
And save a lot of electricity in the process.
To learn about the in-depth science involved in this research, check out this article on the Engineering School’s website.
Media Contact
Article Information
January 2, 2025